Going Deeper - "Tech"
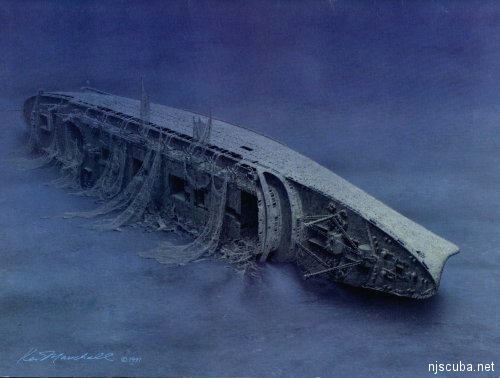
If Nitrox will not take you deeper than air, what will? I touched on a couple of possibilities previously - Trimix and Heliox, but I did not explain what they are, or how they work. I'm not a "Tech" diver; I'm not interested in going deep enough to actually need such gas mixes, and I've never taken a formal class in such things. But I do have an engineering degree, curiosity, and half a brain.
This next section should be considered nothing more than "thinking out loud." It is in no way a reference on mixed-gas technical diving, it is just a bunch of hypothetical calculations that have nothing to do with the real world.
How Not To
From the previous section on Nitrox, we can see that higher Nitrox mixes have shallower usable depths. The "least" and therefore deepest Nitrox mix is good old air - EAN21, 21% oxygen and 79% nitrogen. Using the Nitrox Mix Calculator and a maximum safe PO2 of 1.4 ATA, we calculate that the maximum usable depth for air is 187 feet, which we will round off to 190 feet. At 190 feet, your PN2 on air is 5.3. In other words, although you are safe from oxygen toxicity, you are also probably narc'ed out of your skull, and very likely to do something stupid and get yourself killed.
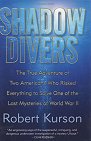
You could also use a maximum PO2 of 1.6 instead of 1.4 in these calculations; I am being conservative here.
There was a time before such limits became known and accepted that divers routinely exceeded these figures, diving to 250 feet ( PO2 = 1.8 ) and well beyond on air, the only gas available. The dangers of "deep air" were well known, and accepted as part of the game. More than a few died. Since the advent of Trimix and modern technical diving protocols, few people dive this way any more.
To go deeper than 190 feet without risking oxygen toxicity, we need to reduce the fraction of O2 in our mix. First, we can try what might be called hypoxic Nitrox: reducing the oxygen content of air to EAN15, for example. Plugging 15% O2 into the calculator gives a max depth of 275 feet. However, the PN2 of this mix at that depth is about 7.9, almost double the recommended limit. You would probably be completely incapacitated by this - i.e. dead. So simply replacing oxygen with nitrogen is not a workable solution.
There is also another problem with any such hypoxic ( < 21% O2 ) mix. The maximum safe partial pressure of oxygen is the well-known 1.4 ATA - the most oxygen our bodies can safely cope with. But what is the minimum amount of oxygen we can tolerate? This is not a subject that comes up much for divers, since we generally operate at increased pressures. It is, however, of major importance to mountain climbers and aviators, who work in reduced-pressure environments with reduced oxygen pressures. Oxygen ATAs as low as 0.16 may result in minor altitude sickness, but most people can still function, perhaps at a reduced level. Oxygen levels below 0.16 ATA are more difficult, especially when under physical stress, whereas at ATAs around 0.10, blackout is swift and certain.
The terms and units used in this discussion are probably unfamiliar to most people, and warrant some explanation:
Pressures are expressed in ATA - ATmospheresAbsolute. 1 ATA equals sea level air pressure ( or 14.7 psia. ) A depth of 33 feet equals 2 ATA, etc.
Gas Fraction is the amount of a gas in a mixture of gases, expressed as a decimal or percentage. For example, the gas fraction of oxygen in air is 0.21, or 21%. This is written FO2 or Fraction of O2, and is independent of pressure or depth.
Partial Pressure is that part of the total pressure that is exerted by a component gas in a mixture. Partial Pressure is equal to the gas fraction times the total pressure of the mixture. For example, the Partial Pressure of oxygen in air at 33 feet ( or 2 ATA ) is 0.21 x 2 = 0.42. This is written PO2, and varies with total pressure or depth.
Concepts such as oxygen toxicity are explained in the section on Nitrox.
Mountain climbers and pilots avoid this problem by going on pure oxygen at altitude. Divers can get around it more easily: just submerge. At a depth of 33 feet or 2 ATA, the partial pressure of oxygen of an 11% mix is 0.22 ATA - roughly the same as sea level. You would not want to use such a low O2 mix for any period of time at the surface, but at even 15 feet it is safe enough. At the surface, you can just breathe the free air, or use what is called a "travel mix." Sometimes, a decompression mix can double as a travel mix.
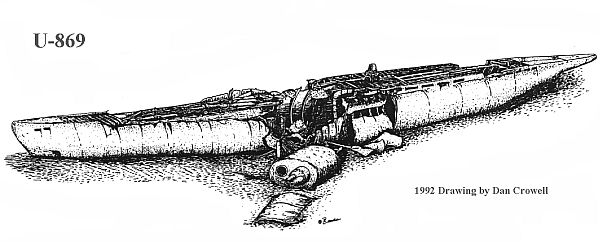
Pick a Gas, Any Gas
What is really needed to go deeper is some way to adjust the oxygen content of the breathing gas, and simultaneously reduce the nitrogen content as well. The obvious solution is to dilute both with a third gas, but which one and how much? First, let's think about which gas to use. Such a gas would need the following properties:
- it should be biologically inert, or at least not poisonous
- it should be non-combustible ( !!! )
- it should be light ( low molecular weight ) and quickly diffuse in and out of the body
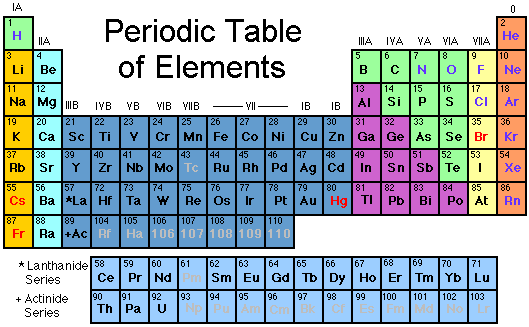
Looking at the Periodic Table, we find 11 'atomic' gases, signified by blue text. That's quite a few to choose from, but as we will soon see, most of them are unsuitable.
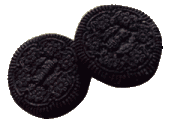
The first gas in the table is hydrogen H. Hydrogen is highly combustible, especially under pressure mixed with oxygen. Experiments with hydrogen mixes (Hydrox) also revealed LSD-like effects at depth.
Nitrogen N and oxygen O are the two gases we are trying to reduce, and so are of no interest. In the yellow column are the two 'Halide' gases: fluorine F and chlorine Cl. Both are highly corrosive and poisonous in gaseous form; chlorine was the major constituent of deadly mustard gas in World War I. ( Chlorine is also one of the constituents of sea salt NaCl. )
The orange column contains the "Noble" gases helium He, neon Ne, argon Ar, krypton Kr, xenon Xe, and radon Rn. The Noble gases are so-named because they generally do not combine with other 'non-noble' elements - they are nearly chemically inert, especially the lighter ones. This is part of the reason they are gases, and why they would appear to be good candidates for our purpose. However, argon and everything below it in the table are all heavier than air. This means that they would tend to pool in the bottom of the lungs, which could lead to asphyxiation - bad. Despite being mostly inert, all the heavier Noble gases, including neon, also turn out to be narcotic at depth. Finally, radon is radioactive. All the noble gases are also quite rare and expensive, especially the heavy ones.
Out of 11 candidates, we are down to one.
But wait, there are other gaseous compounds, thousands of them. What about other 'molecular' gases? Unfortunately, most of them can be eliminated as well. High concentrations of carbon dioxide hinder our breathing reflex, while even low concentrations of carbon monoxide are toxic; both would lead to underwater blackout if used in a breathing mix. Water vapor is strictly avoided in breathing mixtures because it would cause regulator icing. In truth, I don't think many other gases have been studied. Fluorocarbons ( like Freon ) and hydrocarbons ( like propane ) are typically not even breathable at normal pressures.
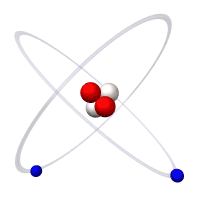
That leaves just helium, the lightest of the Noble gases, shown here at right. Fortunately, though, helium would appear to be near-perfect for our purposes: it diffuses quickly out of our tissues during decompression, it is inert, and has no appreciable biological action - there is no such thing as helium narcosis. ( That's not quite true, and oxygen is also slightly narcotic at depth, but neither is anywhere near as bad as nitrogen. ) By virtue of its low density, helium also reduces the work of breathing under pressure.
The drawbacks of helium are that it makes your voice unintelligible, and it is an excellent conductor of heat. This makes you very cold just from breathing a helium mix, and also requires the use of a separate drysuit-inflation gas, typically argon or air. Although it is the second most common element in the universe after hydrogen, here on earth, helium is rather difficult to come by. For many years, the US government controlled the world's entire supply as a strategic material, and helium was simply unavailable. That is no longer the case today, but it is still expensive. Helium is used in two deep-diving breathing mixes: Trimix and Heliox.
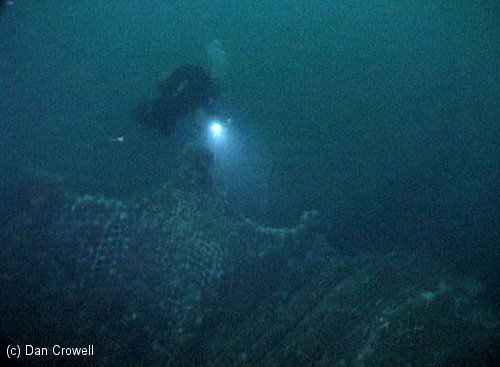
Getting Down - Trimix & Heliox
A mixture of oxygen, nitrogen, and helium is known in diving circles as Trimix, and it was first experimented with in the 1980s by cave divers. But how much of each gas should we use? That depends on your target depth. Remember, we are aiming for a PO2 of 1.4 ( or less ) and a PN2 of 4.0 ( or less. ) Going back to the Nitrox Mix Calculator, we input a PO2 of 1.4 and a depth of 240 feet ( which is coincidentally the max depth on the Andrea Doria ), and get an oxygen fraction of 17%. Good - this mix will even be breathable on the surface. The next step is to determine what fraction of nitrogen will give us a PN2 of 4.0. Since the pressure equations are independent of the actual gas, we can use the PO2 and FO2 calculations to calculate the required FN2. Putting in 4.0 in the PO2 slot, and a depth of 240, we calculate an FN2 of 48%. ( Plus or minus 1% is close enough. )
The remaining calculation is simple:
FO2 | + | FN2 | + | FHe | = | Total |
17% | + | 48% | + | ? | = | 100% |
Therefore, for this dive, the helium fraction of the bottom mix should be 35%. In diving parlance, this is known as Trimix-17-35. Similarly, for a dive to 400 feet, we could calculate a mix of 11% O2 + 30% N2 + 59% He. Trimix-11-59 would definitely be one of those hypoxic mixes that you would not use on the surface. So how deep can you go? Mathematically, you could keep increasing the helium fraction forever, but in the real world, other biological factors come into play to limit diving depths. To be more conservative, one might do the calculations using a lower maximum PN2, say 3.2 ATA. This would lead to a lower fraction of nitrogen, and a higher fraction of helium. A mix like this might also be used to shorten decompression times on non-saturation dives.
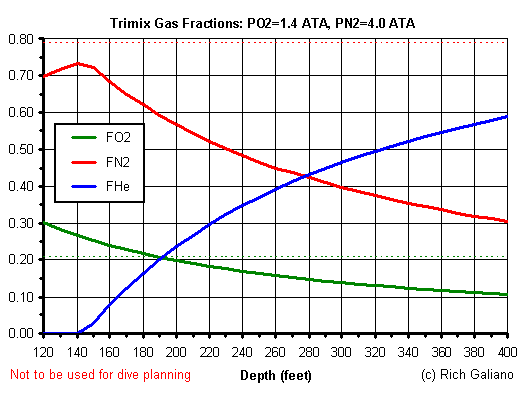
This is a single set of curves calculated for the limiting O2 and N2 gas pressures. While these may be considered "ideal" Nitrox mixes in that they follow both the oxygen and nitrogen limits for any depth, they are still not necessarily the best formulations for a particular dive. Such things may depend on expected dive profiles, personal experience, and personal preferences, and a number of "non-ideal" formulations are commonly used for specific purposes. It all gets very complicated. For example, a "non-ideal" formulation may yield nearly the same results at a significant cost savings, while higher helium fractions may shorten overall decompression times on certain dive profiles.
Incidentally, all of the gas-mixing theory presented here is derived from a single equation:
PX = ( D / 33 + 1 ) * FX
where: | units | precision | |
PX = example: | target partial pressure of gas X 1.4 for oxygen (max) | ATA | +/- 0.1 |
FX = example: | gas fraction of gas X 0.21 for oxygen in air | none | +/- 0.01 |
D = example: | depth ( salt water ) do you really need an example? | feet | +/- 10 |
Since everything having to do with decompression is approximate anyway, values are usually rounded-off to the precisions shown. You can easily calculate more decimal places, but you would be fooling yourself to believe them.
Some easy algebraic manipulations yield the following useful forms:
FX = PX * 33 / ( D + 33 )
D = 33 * ( PX / FX - 1 )
The Nitrox Mix Calculator merely implements these formulas for the special case of a two-gas mix of oxygen and nitrogen, with a few extra bells and whistles.
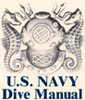
Download the Navy Dive manual
948 pages, PDF, 47MB, Free !
You can't live without the oxygen in the mix, but you can live without the nitrogen. If nitrogen is bad, why not replace it all with helium? A two-gas mixture of oxygen and helium is called Heliox. Heliox was pioneered by the US Navy in the 1930s, and it is still their preferred deep-diving gas. The mathematical calculations for Heliox are much simpler than for Trimix. Going back one more time to the Nitrox Mix Calculator, for our Doria dive, we input a depth of 240 feet and a PO2 of 1.4, which gives the same 17% oxygen fraction as before. Omitting the nitrogen means we must use a helium fraction of 83%. That gives us almost 7 atmospheres of helium at 240 feet, but since helium is harmless ( mostly ) that doesn't matter! Not only that, the light little helium molecule off-gases much faster than nitrogen, which can speed decompression.
Wow, helium is like magic ! Hold on a second, though, helium is not cheap; Trimix and Heliox fills are expensive, and shops that do them are few and far between. Also, gas consumption on open-circuit scuba gear at these depths is horrendous - you will be blowing out great volumes of the precious stuff, which means you will have to start out with great volumes of it if you expect to have any meaningful bottom time. This is where closed-circuit rebreathers really come into their own - they maximize the amount of diving that can be done on a small amount of gas. The trade-off is a great deal of additional complexity and risk, not to mention the five-figure price tags of such devices. If you think diving is expensive, technical diving is very expensive.
But more important than the cost of the dives is your own safety and well-being. The helium may get you down to the wreck without narcosis, but what is going to get you back? Getting down is the easy part; getting back where the danger really lies. Decompression diving is far more unforgiving than recreational diving, and technical diving with helium mixes is especially so. Helium is a "fast" gas - body tissues become saturated with it much quicker than with nitrogen, and it comes out of solution much faster than nitrogen. Strict decompression procedures are required with helium mixes to avoid bubbles and "helium bends." Of course, with mixes containing nitrogen, nitrogen bends are also a possibility, and decompression schedules must take both gases into account.
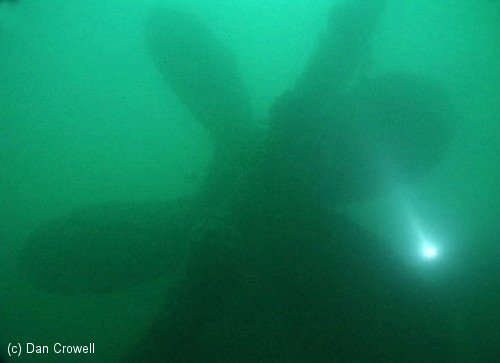
Getting Back - Decompression
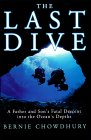
In decompression, the goal is to remove the "inert" gases - nitrogen and/or helium - that have accumulated in your body as safely and quickly as possible. ( Oxygen is actively consumed by the body, and so does not accumulate in the tissues to any great degree. ) Without proper decompression, dissolved gases may bubble out of the tissues, causing the "bends" or DCS - Decompression Sickness. Symptoms of DCS can range from "skin hits" - minor rashes - to pain, paralysis, blindness, permanent disability, and death. The Last Dive by Bernie Chowdhury is a manual on how to get bent, and good cautionary reading for any aspiring tech diver. Yikes!
Mix %O2 | Max Depth PO2 = 1.6 |
Air | 218 ft |
36% | 114 ft |
50% | 73 ft |
70% | 42 ft |
80% | 33 ft |
100% | 20 ft |
While it is possible to decompress on the same mix that you used on the bottom, for anything more than minor decompression this is usually inefficient, resulting in overly long hang times. Therefore, to speed off-gassing of inert gases, special-purpose decompression mixes are used. These are typically high-oxygen "technical" Nitrox mixes, and even pure oxygen. Such mixes have definite depth limits, as shown in the table at right. Of course, even carrying a high O2 mix with you on a deep dive adds a certain amount of risk, as more than one narc'ed diver has accidentally switched to his deco mix on the bottom. The result is usually fatal. ( An interesting side note: for decompression from a Heliox dive, plain old air is as good a mix as anything, since it contains no helium! )
For extreme dives, a diver may carry several different decompression mixes for use at different depths during the ascent, hence the term "staged decompression." For a complex decompression, the collection of bottles ( travel gases, bottom gases, deco gases, suit inflation, etc ) can become quite a burden. The sequence of depth, time, and gas combinations that must be carried out during an ascent from a technical dive is known as a decompression schedule. A planned decompression schedule ( along with several emergency alternates ) is usually worked out in advance using computer dive simulation software and then carried on a dive slate during the dive. Alternatively, it can be done on the fly by a dive computer ( if you are a fool. )
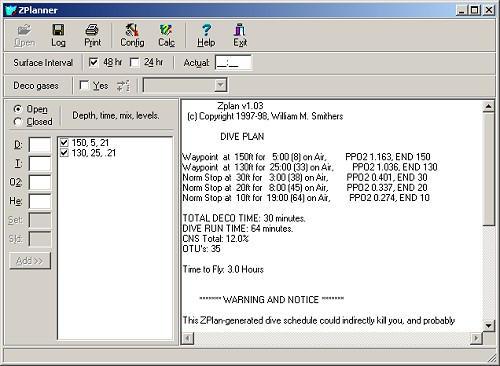
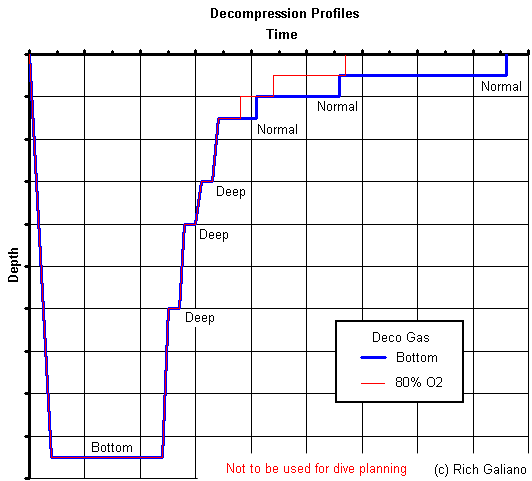
Deep stops utilize bottom gas; Normal stops utilize deco gas ( if any. ) Using 80% Nitrox for decompression yields obvious savings in decompression time.
The bottom gas in this example could be either air or Trimix - it makes little difference in this case. Either way, the diver incurs a substantial decompression obligation - a direct ascent to the surface would almost certainly land you in the recompression chamber, if it did not kill you outright. For Trimix, the decompression schedule must account for both gases. The initial deep decompression stops are governed by helium, which off-gasses quickly. Slower nitrogen becomes the driving factor in later shallower stops. On air, the deep stops are not obligatory, but are good practice. The numbers have been deliberately omitted.
The Oxygen Window theory states that decompression is most efficient at PO2s of 1.0 and higher. Breathing oxygen at such high partial pressures leads to two complications:
Pulmonary Oxygen Toxicity (PO) occurs after several hours of continuous exposure at levels as low as 0.6 ATA. The lung tissues become damaged and enflamed, causing asthma-like reactions and pain. PO toxicity is not a serious problem for most divers, since it is difficult to expose oneself to oxygen long enough for this to become a real problem.
Central Nervous System Oxygen Toxicity (CNS) is a much more serious concern. Over much shorter durations than for Pulmonary Oxygen Toxicity, high levels of oxygen exposure ( > 0.9 ATA ) attack the central nervous system, resulting in visual disturbances, ringing in the ears, dizziness, mood swings, convulsions, and finally coma. Managing CNS Oxygen Toxicity is a part of any technical dive plan.
NOAA Recommended Maximum Oxygen Exposures
( Central Nervous System Oxygen Toxicity )
PO2 | single exposure | 24 hour exposure | ||
ATA | minutes | % per minute | minutes | % per minute |
0.6 | 720 | 0.139 | 720 | 0.139 |
0.8 | 450 | 0.222 | 450 | 0.222 |
1.0 | 300 | 0.333 | 300 | 0.333 |
1.2 | 210 | 0.476 | 240 | 0.417 |
1.4 | 150 | 0.667 | 180 | 0.556 |
1.6 | 45 | 2.222 | 150 | 0.667 |
For example, the following CNS O2 toxicity figures approximate the dive profile above:
Stage | Time minutes | FO2 % | PO2 ATA | %CNS single | %CNS 24 hrs |
bottom | 23 | 21% | 1.4 | 15.34% | 12.79% |
deco | 2 | 21% | 1.2 | 0.95% | 0.83% |
deco | 2 | 21% | 1.0 | 0.67% | 0.67% |
deco | 2 | 21% | 0.8 | 0.44% | 0.44% |
deco | 8 | 80% | 1.6 | 17.78% | 5.34% |
deco | 16 | 80% | 1.4 | 10.67% | 8.90% |
deco | 31 | 80% | 1.0 | 10.32% | 10.32% |
Total: | 56% | 39% |
As usual, everything is approximate, and the result is rounded off to the nearest 1%. At 56% of the single-dive exposure limit, this dive is well within NOAA recommendations. A repetitive dive of the same profile would yield 78% of the 24-hour limit, also within NOAA recommendations. However, a third dive would go over 100% of the 24-hour limit.
Here's a way to hit the CNS O2 limit in one dive: 36% Nitrox for 45 minutes at 114 feet, 12 minutes decompression, 63 minute total run time. This is a reckless dive profile, and yet it is entirely possible with typical equipment and a recreational Nitrox C-card. This might even be considered an ordinary dive by some people who don't fully understand what they are doing!
Mixing It Up
Trimix and Heliox are normally made by blending quantities of pure oxygen, nitrogen, and helium. This is a complex, time-consuming, and expensive process. To simplify and cut costs, several short-cuts have been devised.
Heliair is a poor man's Trimix, made by adding helium to ordinary air. It can be made relatively cheaply with just a bottle of helium and an air compressor. This also avoids any need to handle pure O2. Heliair is inherently hypoxic and is sub-optimal at any depth compared to "ideal" Trimix. Normoxic Trimix is a sort of "Trimix Junior" that is limited to oxygen fractions of 21% or higher, with a resulting depth limit of 190 feet. Normoxic Trimix can be made in much the same way as Heliair, using banked Nitrox, although it does not have to be made this way.
While neither of these mixes is optimal, both can extend depths and/or bottom times. They can also be used for non-technical no-decompression recreational diving ( as can real Trimix, although that would be a very expensive way to see the Mohawk.) For these uses, helium probably adds a degree of cost, complexity, and risk that is not worth the extra few minutes. Heliair and Normoxic Trimix are generally not considered to be full technical training.
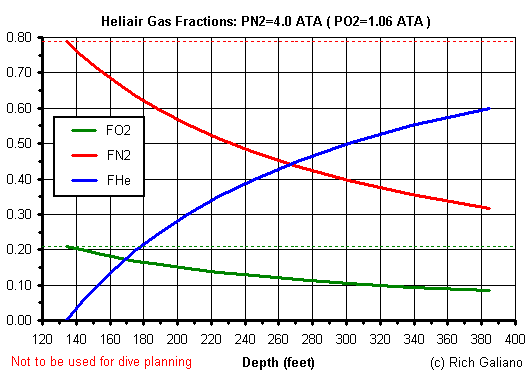
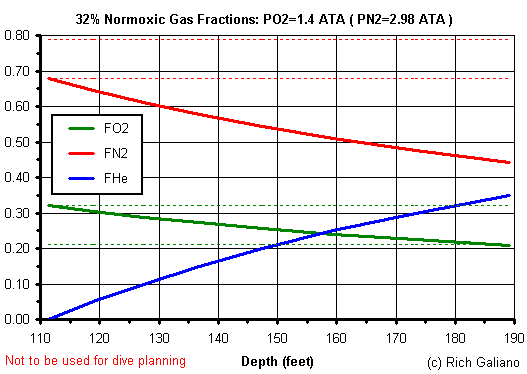
Comparison of gas mixes at 190 feet
Depth = 190 ft | PO2 | PN2 | FO2 | FN2 | FHe |
Air | 1.4 | 5.3 | 0.21 | 0.79 | 0.00 |
Trimix - best mix | 1.4 | 4.0 | 0.21 | 0.59 | 0.20 |
Trimix (He+32% Nitrox) | 1.4 | 3.0 | 0.21 | 0.44 | 0.35 |
Heliair (He+Air) | 1.1 | 4.0 | 0.16 | 0.59 | 0.25 |
Heliox | 1.4 | n/a* | 0.21 | 0.00 | 0.79 |
Final Words
That covers much of the basic theory of technical diving. But doing the math is a far cry from actually doing the dives. While some of the deep-diving procedures can be taught, a great deal more comes from experience: knowing what to do, knowing what to do when things go wrong, and above all, not panicking when things go very wrong. Rushing into technical diving is the surest way to get hurt or killed - it takes years of slow steady advancement to develop true proficiency. I know a lot of great technical divers, and none of them got where they are overnight.
At this point, you may have noticed that I have not actually given numbers for bottom times, run times, decompression schedules, etc. I am not going to. As I said at the beginning, this is just thinking out loud. If you really want to get into technical diving, then sign up for a Technical Diving ( not DIR ) course - the first step on the long path to the Doria.
Me, I'm happy just splashing around in the shallows!
DIR
Cave divers deride 80% Nitrox as "stroke mix" ( "stroke" is their derogatory term for anything they don't like ) and prefer 100% O2. That might be fine in a nice calm cave, but in the unpredictable open sea, the extra depth range of 80% makes it the deco mix of choice. Some wreck divers even prefer 70%, since you can "get on it" as deep as 40 feet if need be. While 70% or 80% may not have as great an effect as pure O2, it is much safer for open-water divers, who are unlikely to need the same extreme decompression as cave divers anyway.
Cave divers can drop all their multiple stage bottles and other junk on the way in, and pick it all up on the way back. This would be an extremely foolhardy practice for a wreck diver because in the open sea you can never be absolutely certain that you will come back the same way you went. Most non-extreme technical wreck diving can be done with a single easily-carried stage bottle, with a single decompression gas, and 80% is it. And you never let go of your stage bottle - read Chowdhury's book if you don't believe me.
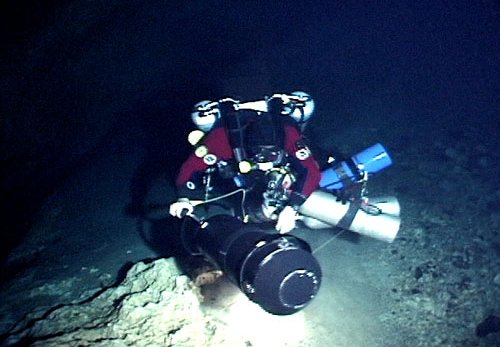